Novel medical imaging technology visualizes disease-related impairments, enabling clinicians to diagnose disorders at their earliest stages and to track the success of treatment with a precision never before possible. Hence, medical imaging technology development will save lives and alleviate suffering on a vast scale.
Director's Message
Welcome to the Advanced Imaging Research Center (AIRC) at UT Southwestern!
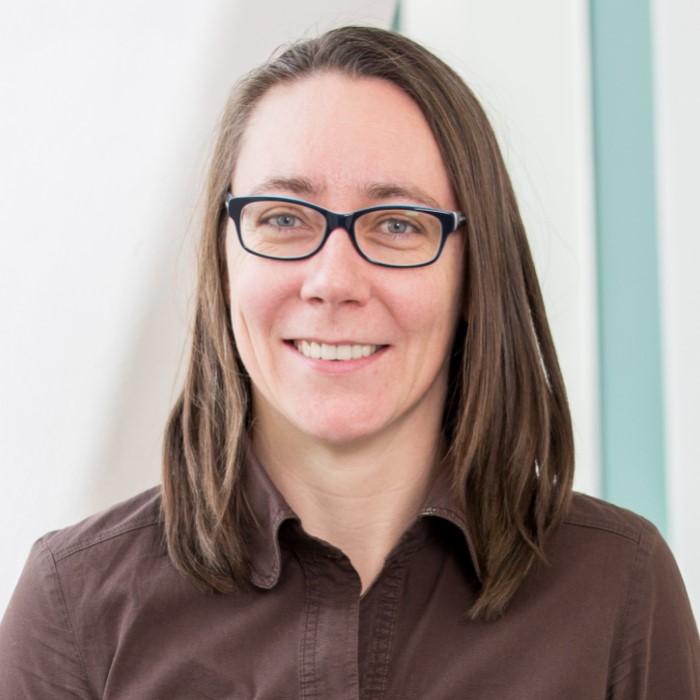
Director, AIRC
Biomedical Imaging, a key diagnostic method that visualizes the internal structure, function, and metabolism of the human body, provides essential information about various diseases, as well as their progression and therapy response. In vivo imaging also delivers instrumental information for basic and preclinical research.
AIRC researchers develop innovative imaging technology to push the current boundaries of biomedical imaging with respect to spatial and temporal resolution, functional and metabolic specificity, as well as speed and spatial coverage. In collaboration with clinical and basic science researchers, these novel technologies are evaluated, refined, and finally translated into clinical diagnostic and clinical, pre-clinical, and basic science research.
The AIRC supports the research of more than 120 PIs across the UTSW campus and in the North Texas research community. It allows access to all imaging instrumentation, providing data acquisition and analysis services. Collaborators and core facility users of the AIRC seek to advance basic understanding, diagnostic techniques, and treatments for a wide range of diseases of the brain, body, and musculoskeletal system. Respective research includes cancer, diabetes, obesity, Alzheimer's, schizophrenia, depression, autism, attention-deficit hyperactive disorder (ADHD), inborn genetic disorders, and diseases of the heart, lung, and liver.
Events
AIRC Annual Symposium & Training
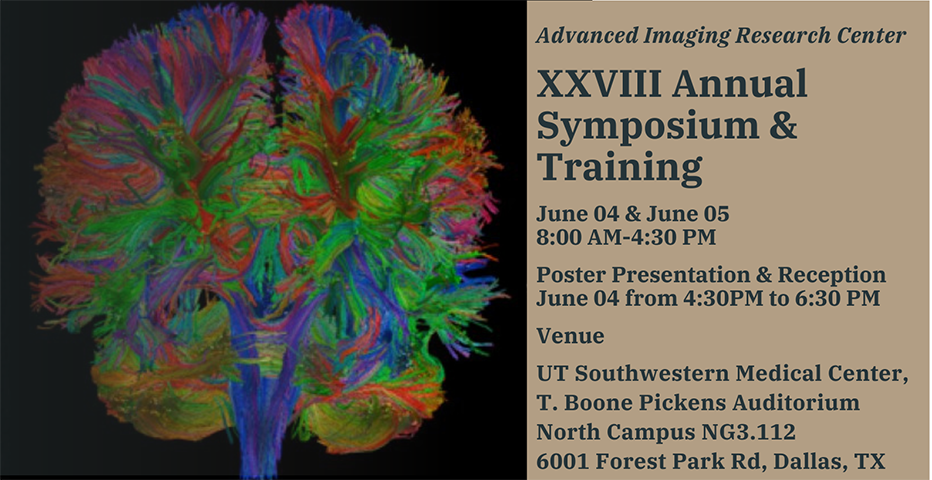
We are excited to welcome you to the AIRC Annual Symposium. Please register by clicking "Start now" on the bottom left of the graphic on the registration page.
2023
We are excited to welcome you to the pre-clinical MRI core opening symposium!
The event is free, but registration is required.
Preclinical MRI Symposium
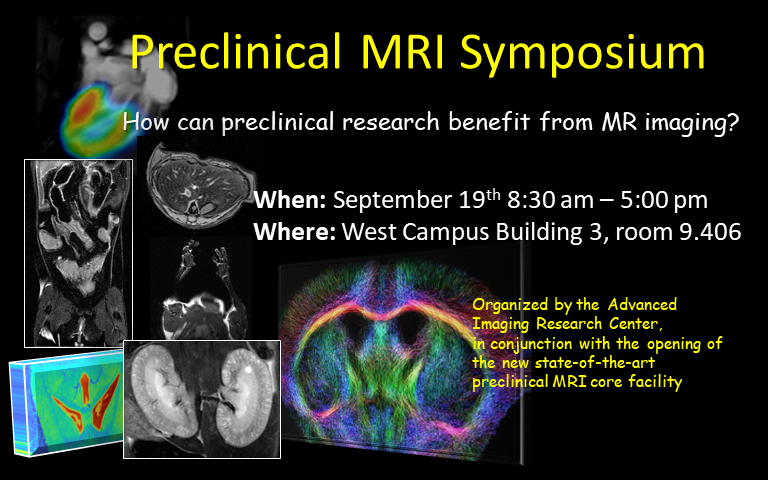
How can preclinical resesarch benefit from MR imaging?
When: September 19th 8:30 a.m. – 5:00 p.m.
Where: West Campus Building 3, room 9.406
Organized by the Advanced Imaging Research Center.
In conjunction with the opening of the new state-of-the-art preclinical MRI core facility.
News
2022 Harry Fischer Award
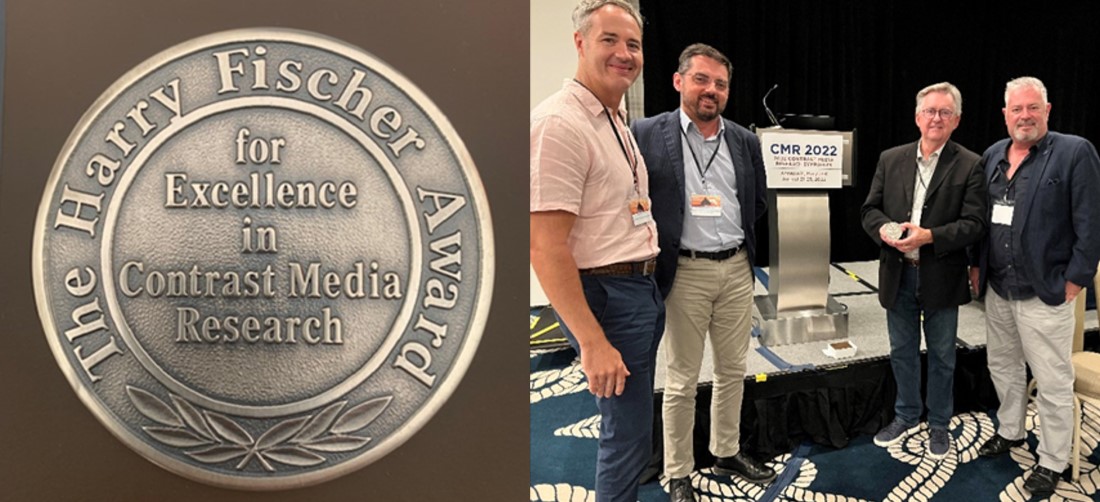
A. Dean Sherry, Ph.D., former and inaugural director of AIRC (pictured second from right), received the 2022 Harry Fischer Award. Presented at the Contrast Media Research Symposium held in Annapolis, MD, August 12-15, 2022. The Harry Fischer Award honors a single scholar who has made outstanding research contributions to the field of contrast agents over a career. Congratulations, Dr. Sherry!
AIRC Seminar Series
Every Tuesday at 9 a.m. in room NE 2M.116 (floor NE 2.5) and virtual via Zoom. To subscribe to email updates, contact Bryan Guardiola via Bryan.Guardiola@UTSouthwestern.edu.